A fusion experiment promised to be the next step in solving humanity’s energy crisis. It’s a big claim to live up to
How close are scientists to developing fusion energy? And what are the roadblocks standing in the way?
Listen 18:51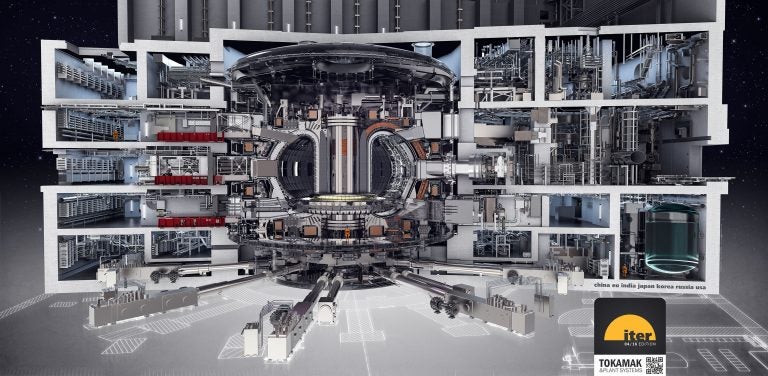
A drawing of the ITER tokamak and integrated plant systems shows the complexity of the ITER facility now under construction in France. (Wikimedia Commons)
This story is from The Pulse, a weekly health and science podcast.
Subscribe on Apple Podcasts, Spotify or wherever you get your podcasts.
Heat, density, time, and a small amount of seawater. In just 20 years, nuclear physicist Mark Henderson says, that simple recipe could power entire cities and help slow the rising tide of climate change for good — if, that is, a few things happen.
“If we put the money, we put the effort, and we put the ingenuity behind it,” Henderson said.
Those latter ingredients, he added, can be harder to come by.
“We would have to be committed to doing that,” Henderson said. “And governments would have to be committed to putting the success of fusion first.”
He hopes the project he’s been working on for the last dozen years will give fusion energy that push.
It’s called the International Thermonuclear Experimental Reactor, ITER for short. Still under construction in the south of France, the huge doughnut-shaped test reactor is often labeled the world’s largest science experiment, and the next step in the journey to fusion energy. (The Latin word “iter” means “way” or “journey.”)

ITER is a product of diplomacy. In the 1980s, two longtime foes, the United States and the former Soviet Union, came together with a common mission: to harness this seemingly magical power source for something other than mutual annihilation. By then, the idea of working together was a more novel concept than fusion power itself.
Henderson has dreamed of building a functional commercial fusion energy reactor since he was 14 years old — another physicist in a long line of boosters who believe fusion energy production is not only achievable, but mandatory, if much of humanity is to outlive the lifestyles of its elite.
And it’s easy to understand why. The potential of nuclear fusion as an energy source is so bright it could blind you. It’s the fundamental reaction that has powered our sun for nearly 5 billion years, and will for about 5 billion more. Compress hydrogen isotopes long enough in a super hot environment and its atoms combine to create a new element: helium. In that conversion, a ton of energy is released. Get the recipe just right (that is, the sun), and the reaction will essentially heat itself.
That means if scientists can figure out a way to reliably produce and sustain nuclear fusion on Earth using elements commonly found in ocean water, virtually unlimited energy could be available at the push of a button — all without the risk of harmful carbon emissions from burning fossil fuels, the variability of wind and solar power, and the potential of meltdowns and radioactive waste from nuclear fission.
The only downside is that it’s all theoretical. Researchers have toiled over fusion power since the 1950s and have yet to build a reactor that can produce more energy than it consumes. That’s where ITER comes in.
“That fusion is a practical source of energy, and that fusion would be commercially viable,” said Steven Krivit, a science writer and publisher of the New Energy Times, an independent outlet where he reports on developments in fusion. “That’s what we were promised.”
ITER’s design wasn’t finalized until 2001, but its approval carried a big punch: a promise to create 10 times more energy than it consumed. At that point, 35 countries had joined the project to split the cost (and benefits) of such an achievement. The final price tag is still up for debate. If you ask ITER, the bill will run around $25 billion. The U.S. Department of Energy puts it at nearly $65 billion.
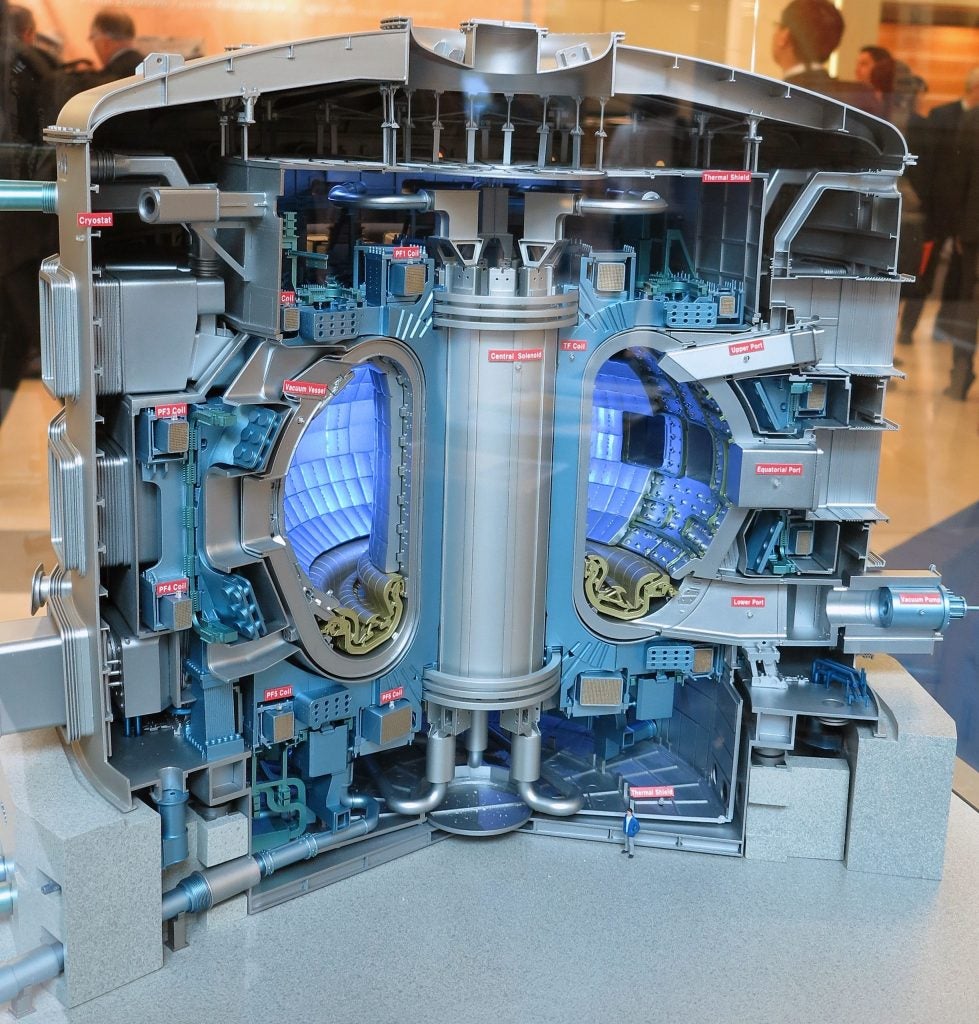
But if ITER were to operate fully as expected by 2035, it would blow all previous fusion reactor designs out of the water in terms of power production. That, ITER often says, is worth the large sums of public cash the investment requires.
But Krivit is skeptical.
“Even though there are many interesting things to learn about fusion research, the only thing that really matters to people — the only thing that really matters to the world — is whether fusion can be a source of energy,” Krivit said. “If the output rate isn’t a lot higher than the input rate, it’s maybe scientifically curious, but it’s practically useless.”
ITER’s made a bold promise: to produce 10 times the amount of energy it consumed. Even though ITER was only a test reactor that would never actually connect to the grid and produce electricity, such a result would be a record-smashing number for fusion reactors compared to its predecessor, a reactor called JET in the U.K. That one couldn’t even break even — meaning it produced less power than it consumed.
ITER’s remarkable energy-production upgrade is thanks to the reactor’s scaled-up design. When it comes to doughnut-shaped fusion reactors called tokamaks, such as ITER and JET, size is a limiting factor. It is ITER’s enormous magnitude, nearly 240 feet tall and weighing 23,000 tons, that allows the organization to make such big claims. And the ITER organization has done so, frequently touting its 10-times power gain number, often called the Q ratio.
But when Krivit needed to double-check some figures for a book he was writing, a closer look at the Q ratio ITER promised revealed something concerning, he said.
“I assumed that everybody knew the rate of power that went into these reactors. But the scientists that I spoke to said, ‘Well, actually, we don’t measure the rate of power that goes into the fusion reactors.’ And I’m going, `What are you talking about?’” Krivit said. “We all thought that the rate of power that you talked about from the JET reactor was a comparison of the power coming out versus the power coming in. And they said, ‘No.’ That power ratio doesn’t compare the rate of power coming out versus power coming in. It only compares the ratio of the power that’s used to heat the fuel versus the thermal power that’s produced by the fuel.”
In reality, the Q ratio only speaks to what happens deep inside the reactor when fusion occurs, not the total amount of energy it takes to run the whole operation, or the actual usable electricity the fusion reaction could produce.
“And I’m thinking, wow, this is a pretty serious misunderstanding that I had, that everybody else that I know had,” Krivit said.
So the first thing he did was get in touch with the scientists at JET, the U.K. reactor that held the previous record for Q. He wanted to find out the ratio for total electrical power input versus total electrical power output had the test reactor been connected to the grid.
“The efficiency is 1%,” Krivit said.
That was far off from the 67% Krivit had previously thought it was. That means if JET were hooked up to the grid, the reactor would lose almost all the power it used to operate.
“My first reaction was ‘Oh, shit.’ There’s a real serious discrepancy here.” Krivit said.
What did all that mean for ITER, and its claim to be able to produce 10 times the power it used?
Everywhere the Q ratio was discussed, others seemed to understand it the same way Krivit had. He pointed to articles in the New York Times, Nature, the Wall Street Journal, and other outlets that conflated the amount of power injected directly into the fuel inside the reactor with the total rate of electrical power to operate ITER.
“All of these news outlets reported that the rate of power that ITER would require to operate was 50 megawatts,” Krivit said.
Fifty megawatts in for 500 megawatts out — that’s how ITER said it would achieve a Q ratio of 10.
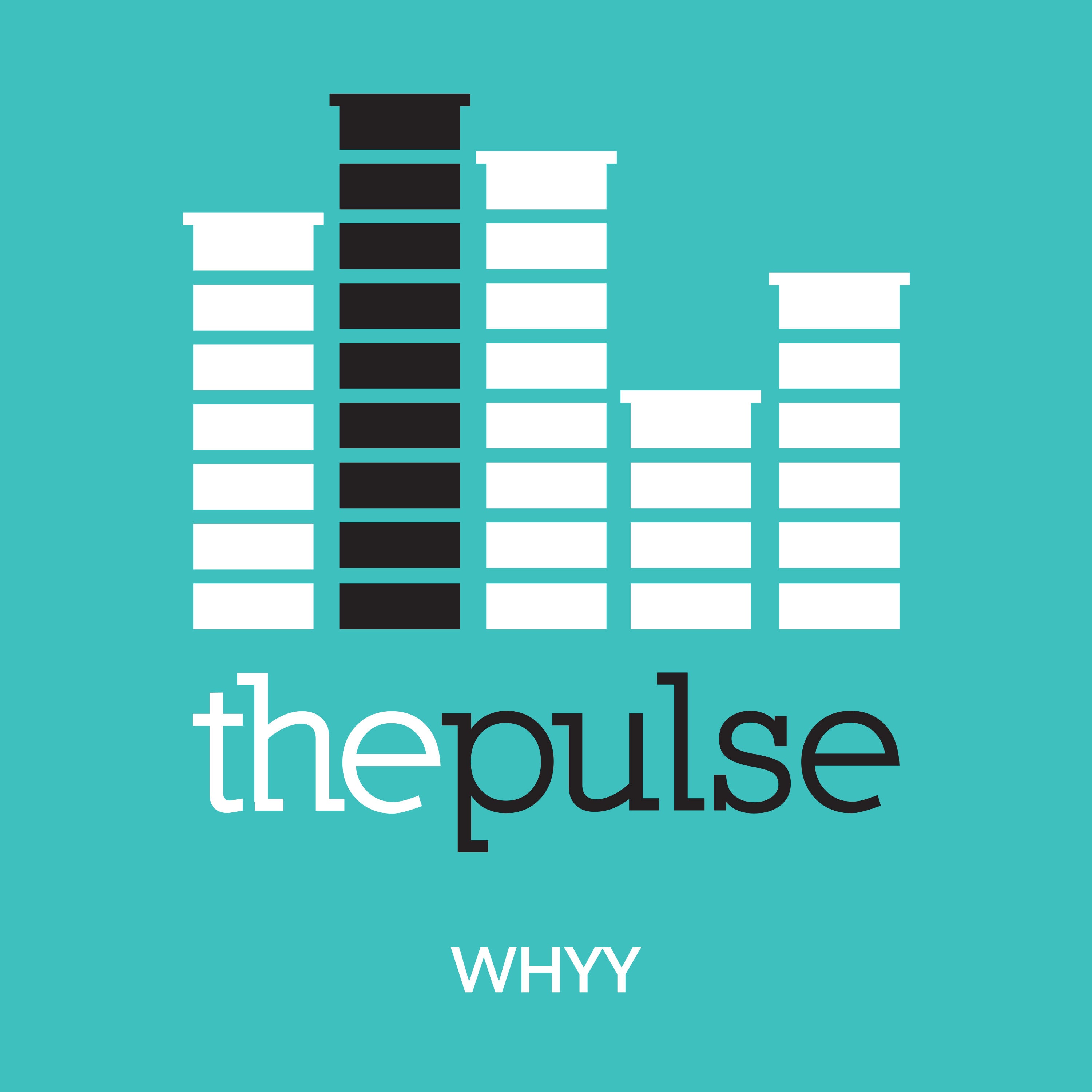
Subscribe to The Pulse
It appears lawmakers might have believed the same. During a congressional hearing on ITER in 2014, California Rep. Eric Swallwell said, “ITER is designed to produce at least 10 times the energy it consumes,” and Texas Rep. Eddie Bernice Johnson added that ITER scientists are “confident it is now possible to actually build a full-scale test reactor that produces far more energy than it uses.” A member of ITER’s governing body who testified at the hearing did not correct these statements.
So what’s the deal? Will ITER actually produce 10 times more energy than it consumes?
Mark Henderson said the answer depends on what you mean by energy.
“One of the confusions on this factor is that there’s really a Q-physics and, to some degree, there’s a Q-engineering.” he said.
According to Henderson, physicists and non-physicists think of Q differently.
“For me to put in 50 megawatts of power, I need to pull from the grid about 150 megawatts. And so an engineer would say, ‘Well, Mark, wait a minute, you know, you’re pulling 150 megawatts from the grid, you convert it to 50 megawatts, it gives me 500 megawatts out, but then those 500 megawatts are going to heat water that turns a turbine that then generates electricity that would put back to the grid roughly 150 megawatts,’” he said. “So from an engineer’s perspective, if ITER was a [commercial] fusion reactor, it would be giving a Q-engineering roughly of about one.”
A Q-physics ratio of 10, but a Q-engineering ratio of one. Henderson doubted whether actual engineers who work on fusion sites correctly understood this difference, let alone the public. But it’s an important difference, nonetheless.
“That means the ITER reactor is effectively a zero-power reactor,” Krivit said. “If the design works as expected, we’re going to get the same rate of power coming out as the power going in. Zero extra power that would be available to use for any practical purposes.”
Krivit noted that if ITER were hooked up to the grid, it would effectively be a massive, expensive, complicated, scientifically remarkable extension of electrical wire. But, of course, ITER was never going to be hooked up to the grid anyway. So that, in itself, wasn’t a huge issue. To Krivit, the issue was one of misrepresentation.
He argued that ITER should have known the Q-physics ratio was going to be misunderstood by the public as Q-engineering; that the organization’s language describing power output and Q was always way too squishy for non-experts to reach any other conclusion than that Q and “fusion power” referred to usable energy rather than heated plasma, especially when those terms were so often cited in the context of “virtually unlimited energy” as a feasible solution to the impending climate crisis that deserved more funding.
Henderson said that if there was confusion, it was all just a misinterpretation. There was no purposeful misrepresentation.
“It is very easy in any field to be misinterpreted. And unfortunately, it’s easier for people to take potshots at fusion, just because [of] the amount of invested money to build a machine,” he said. “People will say, ‘Well, why are you building a fusion reactor compared to, for example, putting the money into a solar field or to a wind field?’ And my answer is to say that, you know, we should be looking at a short-term objective and a long-term objective.”
Still, during a TED Talk in 2019 entitled, “The Dawn of the Fusion Age,” Henderson told an audience, and the internet, that ITER is designed to “have 1 watt going in equals 10 watts out.”
Though that claim is technically true, did the audience of non-physicists know he was referring to directly injected energy and thermal power? Or did they assume he was talking about overall electrical power?
“I don’t like conveying information in a way that can be misinterpreted,” Henderson said. “And I think one of the key issues in communication is to be able to understand who your audience is, and to give that audience enough information to be able to understand what this thing called capital Q is. So obviously, fusion has hurt itself in how the capital Q has been interpreted in the community or in public.”
Henderson said a commercial fusion reactor would need a Q-physics of about 40, four times the Q of ITER, to generate sufficient power to be viable.
So why all the fuss about Q=10 from ITER? The number represents an incredible leap forward in fusion, but a leap nearly 50 years and an unknown sum of money in the making, if things go according to plan — a plan over which there seems to be much confusion.
Michel Claessens was the communications director for ITER for about five years and wrote a book about the project, in which he spoke bluntly about Q.
“People just accepted this. And because it was, let’s say, a good selling argument — voila,” Claessens said with a chuckle. “It went through the system.”
He said it took awhile to correct the representation of Q on ITER’s website.
“This was either too vague or uncorrect. So I had the impression that we were mixing the two arguments. Of course, from a selling point of view, it’s quite attractive. ‘You will produce 500 megawatts from just 50 [megawatts] injected.’ So a gain factor of 10. This was very interesting, very promising,” Claessens said. “So it actually took several years to have this corrected, and I just this morning went through an article published by an outlet and they are still using the same explanation.”
The current head of communications for ITER, Laban Coblentz, isn’t too concerned that ITER’s past power claims will fuel skepticism about future fusion projects. He said the organization does not believe there is any public misunderstanding of the Q ratio, nor does it believe its representation of Q was vague or incorrect.
“I don’t think there is a gigantic public deception or misconception. I think that on this specific thing, if you focus it on something like Q, and you went to the next 100 people outside your house or office building, and ask them about it, they wouldn’t have any idea,” Coblentz said. “But if you [found] some people who know about the project, and you ask them about the significance of ITER, I think they would correctly say that ITER is trying to demonstrate the feasibility of fusion power.”
Even if they didn’t understand that the project isn’t producing usable energy, he added, “They would still be right in their basic assumption, which is that ITER is a groundbreaking science project that is based on paving the way for commercial fusion. It’s still correct.”
Coblentz said that ITER has made efforts in the past to reach out to publications and journalists when it felt the Q ratio was misrepresented, and that despite simplifications made by congressional representatives during hearings, ITER is confident lawmakers correctly understand the goals of the project. He said that the ITER organization believes fusion energy will be a crucial power source in the future, and that ITER is the way to get there.
In the meantime, Steven Krivit has reached out to the organizations who had published the Q ratio without an explanation of what it meant. He’s edited the ITER Wikipedia pages to better explain Q, and personally emailed ITER member organizations from all over the world to get the number changed on their sites.
“And the thing is, nobody has ever disagreed with me,” Krivit said. “All of these people that I contacted at these organizations, nobody’s ever argued with me about the power values, never. They’ve either made changes, or they just left it alone.”
WHYY is your source for fact-based, in-depth journalism and information. As a nonprofit organization, we rely on financial support from readers like you. Please give today.